Conventional mechanical methods of crack analysis presume that cracks form on a material’s two-dimensional surface or that they are planar. Most cracks, including those in common brittle substances like glass, propagate into three-dimensional networks of ridges and other complicated features; superficial planar cracks are merely the tip of the iceberg.
Monitoring this complexity in real time is quite challenging because of the opacity of the material and the speed at which cracks occur. But now that they have a confocal microscope and a Swiss Army knife at their disposal, John Kolinski and his team at the Laboratory of Engineering Mechanics of Soft Interfaces (EMSI) in EPFL‘s School of Engineering have succeeded in doing just that, and they have also found a positive association between material toughness and crack complexity.
Kolinski said, “The energy required to drive cracks has traditionally been considered a material property, but our work yields unique insights into the key role of geometry: namely, that by increasing the complexity of geometric features at the crack tip, a material can be effectively made tougher because more strain energy is required to advance a complex crack than a simple one. This highlights an important gap in the current theory for 3D cracks.”
The researchers’ technique involved cutting an elastomer and four distinct hydrogels into extremely thin slices. The fragile and transparent hydrogels were useful as a stand-in for glass and brittle plastics to help understand how cracks originate in these materials. They were also easy to measure and deform without breaking. Moreover, the elastomer served as a stand-in for substances like silicone polymers and rubber.
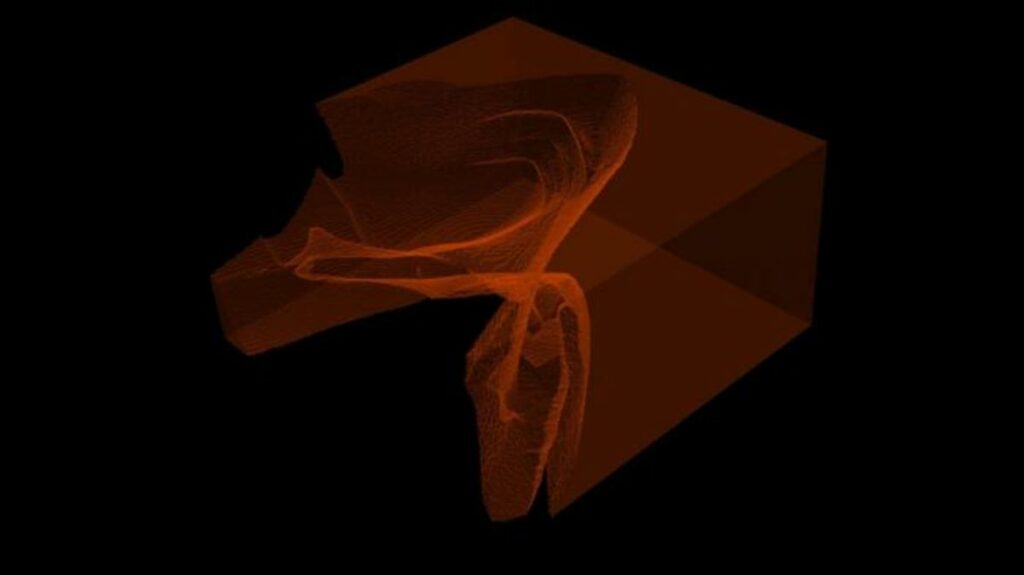
The device’s scissors created geometrically intricate cracks in the hydrogel samples, which were then seen with a state-of-the-art confocal microscope. The Swiss Army knife was used to induce the experimental cracks. A sequence of fluorescent images was created with the confocal microscope using special equipment that the EMSI team constructed to manage sample alignment and loading. These images were then layered to create a distinct, three-dimensional map of each fracture surface.
Kolinski explains, “People have long known that cracks can become complex by looking at fracture surfaces after the fact, but what is lost is the understanding of the loading conditions when the crack emerged, or what forces the sample was exposed to. Our innovative imaging method has made it possible to characterize this relationship rigorously in situ.”
In summary, the investigations showed that the lengths of the crack ends were directly correlated with the strain energy needed to drive the sample cracks. This implies that a 3D crack’s greater geometric complexity creates more fracture surface as it spreads, necessitating higher strain energy to drive it.
In another experiment, the researchers demonstrated how the planar symmetry of a smoother crack was broken as it got closer to a rigid impediment buried in the sample, lengthening the crack tip and requiring more energy to move the crack ahead.
Kolinski says, “The fact that we can isolate how geometric complexity emerges with such an inhomogeneity in the material could motivate new design approaches. Our work also highlights the importance of care in carrying out materials testing, as we now know that any geometric deviation from a planar crack front may lead to a mis-measurement – and potentially dangerous over-estimation – of material toughness.”
Journal Reference:
- Wei, X., Li, C., McCarthy, C., et al. The complexity of crack front geometry enhances the toughness of brittle solids. Nat. Phys. (2024). DOI: 10.1038/s41567-024-02435-x