While early multicellular lineages necessarily started as relatively simple groups of cells, little is known about how they became Darwinian entities capable of sustained multicellular evolution. A new study investigated this with a multicellularity long-term evolution experiment.
Scientists from the Georgia Institute of Technology aimed at evolving new kinds of multicellular organisms from single-celled ancestors in the lab. They selected ‘snowflake yeast’ because all multicellular lineages started small and simple, but many became larger and more robust over time.
The ability to grow large, tough bodies plays a role in increasing complexity, as it requires new biophysical innovations. Their yeast developed over roughly 3,000 generations to produce groupings more than 20,000 times bigger than their ancestor. They grew from being invisible to the naked eye to fruit fly size, with over 500,000 cells. While starting weaker than gelatin, the unique snowflake yeast gained new material qualities to become as strong and resilient as wood.
The scientists saw how their model organism, “snowflake yeast,” started to adapt as multicellular individuals throughout 3,000 generations of laboratory evolution. They showed how snowflake yeast evolved to be physically stronger and more than 20,000 times larger than their ancestor.
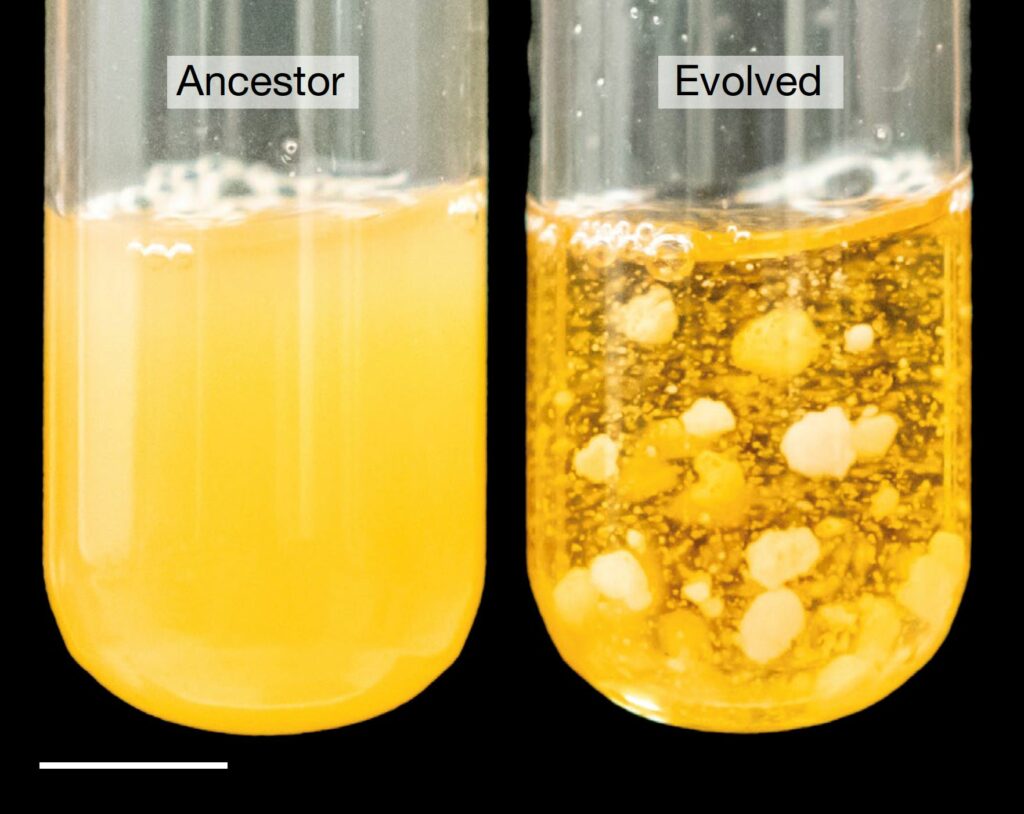
The kind of enormous multicellular life that can be observed with the naked eye requires this kind of biophysical evolution as a prerequisite.
The scientists discovered that the yeast cells grew longer as they tried to understand how the snowflake yeast changed to become bigger. This resulted in fewer cells being packed closely together in each group. The increase of cell-to-cell stress that would often cause the clusters to break apart was slowed down by this cell elongation, allowing the groupings to grow. The size and multicellular toughness, however, should have only slightly increased as a result of this fact alone.
The scientists had to investigate the physical interactions between the cells within the yeast clusters to determine the specific biophysical mechanisms that permitted growth to macroscopic size. The scientists used a scanning electron microscope to image thousands of skinny yeast slices, revealing their interior structure. Since normal light microscopes could not reach the large, densely packed groups, this method was necessary.
Ozan Bozdag, a research scientist and former postdoctoral researcher in Ratcliff’s group, said, “We discovered that there was a new physical mechanism that allowed the groups to grow to this very, very large size. The branches of the yeast had become entangled – the cluster cells evolved vine-like behavior, wrapping around each other and strengthening the entire structure.”
The scientists discovered how to use the biomechanical principle of entanglement by merely choosing organismal size, which ultimately made the yeast around 10,000 times tougher as a material.
Peter Yunker, associate professor in the School of Physics and a co-author, said, “Entanglement has previously been studied in totally different systems, mostly in polymers. But here we see entanglement through an entirely different mechanism — the growth of cells rather than just through their movement.”
When scientists saw the entanglement, they realized how simple multicellular groups evolve. Snowflake yeast is a novel multicellular organism that lacks the complex developmental pathways found in contemporary multicellular creatures. However, the yeast discovered how to manipulate and appropriate cellular entanglement as a developmental mechanism after just 3,000 generations of laboratory evolution.
According to preliminary research, other multicellular fungus also produces highly entangled multicellular organisms, indicating that entanglement is a common and significant characteristic of multicellular life in this branch.
William Ratcliff, associate professor in the School of Biological Sciences and director of the Interdisciplinary Graduate Program in Quantitative Biosciences, said, “I’m excited to have a model system where we can evolve early multicellular life over thousands of generations, harnessing the awesome power of modern science. In principle, we can understand everything happening, from the evolutionary cell biology to the biophysical traits which are directly under selection.”
“By putting our finger on the scale of a single-celled organism’s evolution, we can figure out how they evolved into progressively more complex and integrated multicellular organisms and study that process along the way. We hope this is just the first chapter in a long story of multicellular discovery as we continue to evolve snowflake yeast in the MuLTEE.”
Journal Reference:
- Bozdag GO, Zamani-Dahaj SA, Day TC, Kahn PC, Burnetti AJ, Lac DT, Tong K, Conlin PL, Balwani AH, Dyer EL, Yunker PJ. De novo evolution of macroscopic multicellularity. Nature, 2023. DOI: 10.1038/s41586-023-06052-1