The thermodynamic properties of compounds, for example, aluminum oxide, which is known as obstinate materials since they liquefy at temperatures over 2,000 degrees Celsius. Although, it is difficult to study their properties because few vessels can withstand the heat to contain them, and those that do often react with the melt and contaminate it.
Now, MIT scientists demonstrated a whole new method to study the thermodynamic properties of these hot melts.
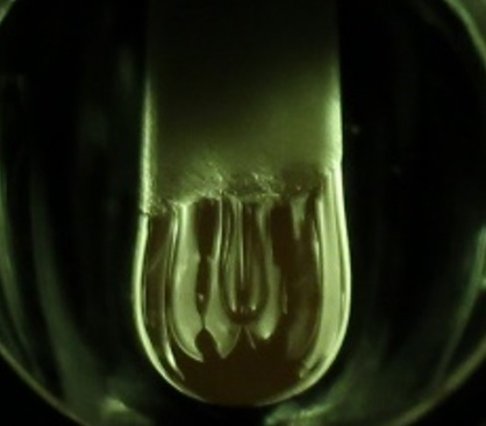
Image: Melody M. Wang
Senior author Antoine Allanore said, “We have a new technique which demonstrates that the rules of electrochemistry are followed for these refractory melts. We have
now evidence that these melts are very stable at high temperature, they have high conductivity.”
Adjusting a thermal imaging (or circular segment imaging) heater all the more regularly utilized for gliding zone precious stone development. Scientists then liquefied an alumina (aluminum oxide) pole and reached the fluid pendant bead that it framed with cathodes, making an electrochemical cell that permitted disintegration of unadulterated, alumina electrolyte to oxygen gas and aluminum compound by electrolysis out of the blue. The aluminum oxide itself fills in as the electrolyte in this electrochemical cell, which works comparatively to water electrolysis.
Brad Nakanishi said, “Decomposition voltage measurements give us direct access to the quintessential thermodynamic property that is chemical potential, also called Gibbs energy. We’ve shown we make electrochemical measurements in a new class of electrolytes, the molten refractory oxides.”
The adjustment in this Gibbs energy, or concoction potential, regarding temperature is known as entropy. Any adjustment in this Gibbs energy, or synthetic potential, as for temperature is known as entropy.
Utilizing this method, four reflected xenon lights focus on the tip of the example, dissolving a fluid bead, which is held to the bar by surface pressure and rapidly cement after the lights are killed. While the bead is melted, the terminals are raised into the drop to finish an electrical circuit, with the fluid alumina itself working as the electrolyte.
Nakanishi said, “That’s something that we have not seen done otherwise, as well, doing electrochemistry in a suspended droplet above 2,000 C. The concentration of the light energy, hot zone, and large thermal gradients present, allows us in a very controlled way to create a situation for stable droplet and electrode contact.”
“It sounds challenging, but the method we’ve refined is straightforward and rapid to operate in practice thanks, in part, to a camera enabling continuous observation of the droplet and electrodes during the experiment.”
Meanwhile, researchers are now able to measure fundamental thermodynamic properties of such a melt.
Allanore said, “In the case of molten alumina, we’ve actually been able to study the property of the cathode product. As we decompose aluminum oxide, to oxygen on one side [anode] and aluminum on the other side [cathode], then that liquid aluminum interacts with the electrode, which was iridium in that case.”
“We can now calculate the thermodynamic property of that alloy, of that interaction, which is something that was never measured before. It was calculated and predicted. It was never measured.”
The nature of the examination is a world-class approach produced for troublesome test investigations of ultra-high temperature responses of fluid metals and fluid oxides, particularly with consideration of electrochemical impedance spectroscopy. The only limitation is the uncertainty of the temperature measurements within a range of plus or minus 10 degrees C.
Future work will only focus on investigating the potential for selectively separating the rare earth oxides.
Nakanishi said, “The research opens up the door to many more candidates for electrolytes that we can use to extract metals, and also make oxygen.”